Table of Contents
Cardiac Action Potential Overview
Cardiac action potentials are rapid shifts in membrane potential that begin with a sudden shift from the normal resting negative membrane potential to a positive potential and finish with an almost similarly rapid shift back to the negative potential.
Specialized Cardiac Excitatory and Conductive system
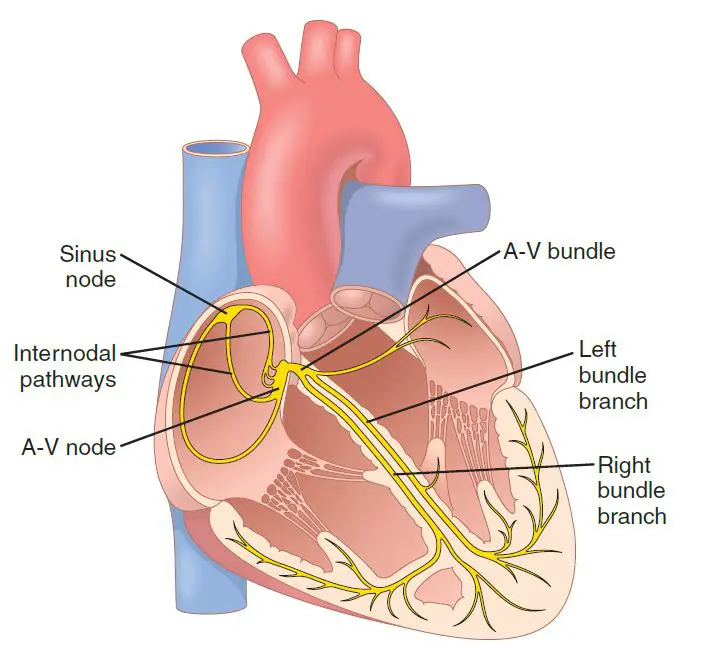
The image showing the heart’s specialized excitatory and conductive system, which regulates cardiac contractions. The sinus node (also known as the sinoatrial or S-A node) generates normal rhythmical impulses. The internodal pathways always conduct impulses from the sinus node to the atrioventricular (A-V) node. The A-V node, which delays impulses from the atria before traveling into the ventricles. The A-V bundle, which conducts impulses from the atrium to the ventricles. Purkinje fibers’ left and right bundle branches, which carry cardiac impulses to all areas of the ventricle.
SA Node (The Sinoatrial Node)
The SA node is a thin, flattened ellipsoid strip of specialized cardiac muscle. Its breadth is about 3 millimeters, length is 15 millimeters, and thickness is 1 millimeter. It is situated in the superior posterolateral wall of the right atrium, just below and slightly lateral to the superior vena cava opening. This node’s fibers almost have no contractile muscle filaments and are just 3 to 5 microns in diameter, compared to 10 to 15 microns for the surrounding atrial muscle fibers. The SA nodal fibers, on the other hand, bind directly with the atrial muscle fibers, so any action potential that starts in the sinus node extends immediately through the atrial muscle wall.
SA Nodal Fibers Automatic Electrical Rhythmicity
Some cardiac fibers are capable of self-excitation, which can result in automatic rhythmical discharge and contraction. This is particularly true of the fibers of the heart’s specialized conducting system, such as the SA node fibers. As a result, the SA node normally regulates the overall heart rate.
Mechanism of SA Nodal Rhythmicity
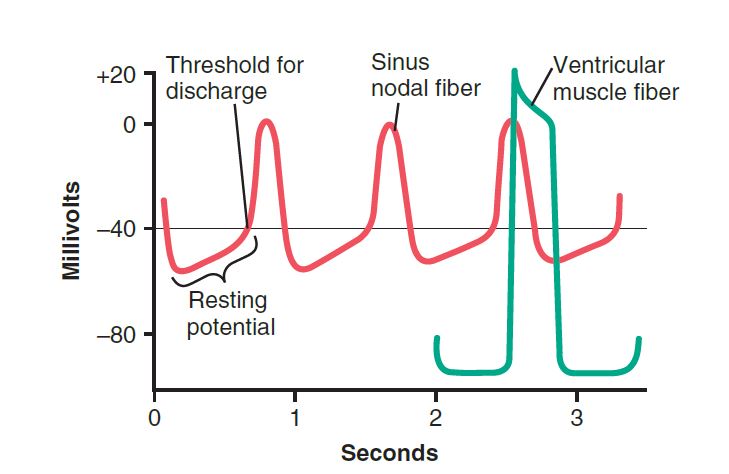
This image showing action potentials collected from within a SA nodal fiber over three heartbeats and a single ventricular muscle fiber action potential for comparison. The “resting membrane potential” of the SA nodal fiber during discharges is approximately -55 to -60 millivolts, while the ventricular muscle fiber is approximately -85 to -90 millivolts. The cell membranes of the SA fibers are normally leaky to sodium and calcium ions, and the positive charges of the entering sodium and calcium ions neutralize some of the intracellular negativity.
Before we discuss the rhythmicity of the SA nodal fibers, first we need to clarify that cardiac muscle has three main types of membrane ion channels that play important roles in causing action potential voltage changes.
Read Cardiac Index
There are three types of sodium channels:
- Fast sodium channels,
- L-type calcium channels (slow sodium-calcium channels).
- Potassium channels.
The rapid upstroke spike of the action potential observed in ventricular muscle is caused by the rapid influx of positive sodium ions to the inside of the fiber, which is caused by the opening of the fast sodium channels for a few 10,000ths of a second. The “plateau” of the ventricular action potential is then triggered mainly by slower activation of the slow ion channels, which lasts approximately 0.3 seconds. Finally, opening potassium channels allows large quantities of positive potassium ions to diffuse outward through the fiber membrane, restoring the membrane potential to its resting level.
After all, the role of these channels in the SA nodal fiber differs because the “resting” potential is much less negative only -55 millivolts in the nodal fiber versus -90 millivolts in the ventricular muscle fiber. At this stage of -55 millivolts, the fast sodium channels have mostly been “inactivated,” which means they are blocked. The reason for this is that if the membrane potential remains less than about 55 millivolts for more than a few milliseconds, the inactivation gates on the inside of the cell membrane that close the quick sodium channels close and remain locked. As a result, only the slow sodium-calcium channels can open (become “activated”), resulting in the action potential. As a result, the atrial nodal action potential develops more slowly than the ventricular muscle action potential. Besides, after the action potential occurs, the potential steadily returns to its negative state, as opposed to the sudden return seen in the ventricular fiber.
Self Excitation of SA Nodal Fibers
Why don’t the SA nodal fibers stay depolarized all the time because of this sodium and calcium ion leakiness? To avoid such a persistent state of depolarization, dual events occur throughout the action potential.
First, the L-type calcium channels become inactive (close) within 100 to 150 milliseconds of opening, while at the same time, a large number of potassium channels activate. As a result, the influx of positive calcium and sodium ions through the L-type calcium channels stops, although significant amounts of positive potassium ions diffuse out of the fiber. Both of these results return the intracellular potential to its negative resting state, resulting in the action potential being terminated. Furthermore, the potassium channels stay open for a few tenths of a second longer, momentarily continuing the flow of positive charges out of the cell, resulting in excess negativity within the fiber; this mechanism is known as hyperpolarization. The hyperpolarization condition initially reduces the “resting” membrane potential to about -55 to -60 millivolts at the action potential completion.
Why isn’t this current state of hyperpolarization permanent? The explanation for this is that over the next few tenths of a second after the action potential ends, more and more potassium channels close. The inward leakage of sodium and calcium ions once again outweighs the outward flux of potassium ions, causing the “resting” potential to rise once more, eventually reaching the discharge threshold at around -40 millivolts. The entire process then repeats itself: self-excitation to trigger the action potential, recovery from the action potential, hyperpolarization just after an action potential has been completed, the drift of the “resting” potential to the threshold, and finally re-excitation to elicit another loop of a cardiac action potential. This process lasts for the rest of a person’s life.
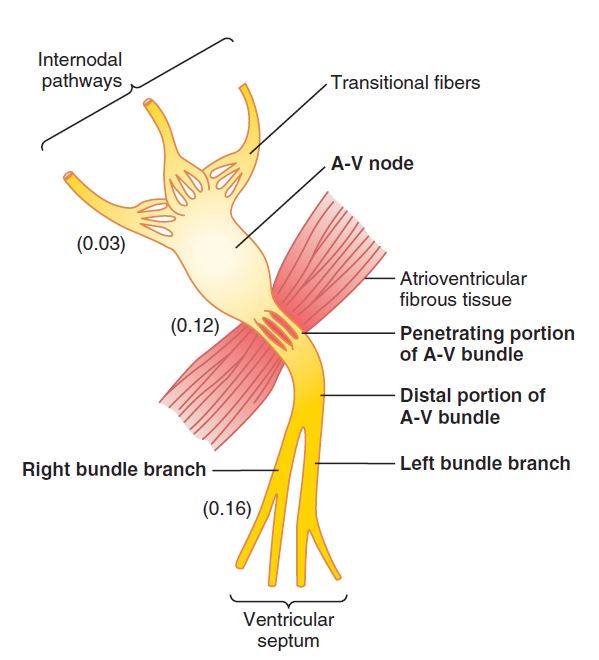
Read Cardiac Glycosides
Internodal and Interatrial Pathways Transmitting Cardiac Impulse Through Atrium
The SA nodal fibers’ ends bind directly to the adjacent atrial muscle fibers. As a result, action potentials from the SA node pass outward through these atrial muscle fibers. As a result, the action potential travels across the entire atrial muscle mass, finally reaching the A-V node. Conduction velocity in most atrial muscles is around 0.3 m/sec, but conduction is faster, about 1 m/sec, in some small bands of atrial fibers. The anterior interatrial band is one of these bands that runs across the anterior walls of the atria and into the left atrium. Besides, three smaller bands curve along the anterior, lateral, and posterior atrial walls before terminating in the A-V node. These are known as the anterior, middle, and posterior internodal pathways. The existence of advanced conduction fibers is the reason for the faster conduction velocity in these bands. These fibers are close to the ventricle’s much faster conducting “Purkinje fibers.”
The AV Node(Atrioventricular node) Delays Cardiac Impulse Conduction from Atrium to the Ventricle
The atrial conductive mechanism is designed so that the cardiac impulse does not move too quickly from the atria to the ventricles; this delay gives the atria time to empty their blood into the ventricles before ventricular contraction occurs. This transmission into the ventricles is mainly delayed by the A-V node and its associated conductive fibers.
The A-V node is situated in the right atrium’s posterior wall, directly behind the tricuspid valve. This image[organization of AV Nodes] showing a diagram of the various sections of this node, as well as its connections to the approaching atrial internodal pathway fibers and the exiting A-V bundle. This diagram also depicts the average time intervals in fractions of a second between the initiation of the cardiac impulse in the sinus node and its subsequent presence in the A-V nodal system. It is important to note that the impulse, after passing through the internodal routes, arrives at the A-V node 0.03 second after leaving the sinus node. The impulse then waits 0.09 seconds in the A-V node before entering the penetrating portion of the A-V bundle and passing through the ventricles. A final delay of 0.04 seconds occurs primarily in this penetrating A-V bundle, which is made up of several small fascicles that move through the fibrous tissue that separates the atria and ventricles.
As a result, the aggregated delay in the A-V nodal and A-V bundle device is approximately 0.13 seconds. This delay, combined with the initial conduction delay of 0.03 seconds from the sinus node to the A-V node, results in an overall delay of 0.16 seconds before the excitatory signal enters the ventricle’s contracting muscles.
Importance and Causes of Slow Conduction
Slow conduction in the transitional, nodal, and penetrating A-V bundle fibers is caused primarily by a decline in the number of gap junctions between successive cells in the conducting routes, resulting in significant resistance to excitatory ion conduction from one conducting fiber to another.
As a result, it is easy to understand why each succeeding cell is slower to be excited.
Rapid Transmission in the Purkinje Fiber in Ventricles
Special Purkinje fibers attach the A-V node to the ventricles via the A-V bundle. Without only the initial portion of these fibers that penetrate the A-V fibrous barrier, their functional properties are opposed to those of the A-V nodal fibers.
They are very large fibers, much larger than average ventricular muscle fibers, and they propagate action potentials at a velocity of 1.5 to 4.0 m/sec, which is approximately six times that of regular ventricular muscle and 150 times that of certain A-V nodal fibers. This velocity allows for nearly instantaneous propagation of the cardiac impulse across the remaining ventricular muscle.
Purkinje fibers’ rapid propagation of action potentials is thought to be triggered by a very high degree of permeability of the gap junctions at the intercalated discs between the successive cells that make up the Purkinje fibers. As a result, ions are quickly transferred from one cell to the next, increasing transmission velocity.
Purkinje fibers often have a low number of myofibrils, which indicates they contract very little or not at all during impulse transmission.
Conduction Through the A-V Bundle is One-way
The failure of action potentials to migrate backward from the ventricles to the atria, even in abnormal states, is a unique feature of the A-V bundle. This property prevents cardiac impulses from re-entering the ventricles through this path, allowing only forward conduction from the atria to the ventricles.
Besides that, except for the A-V bundle, the atrial muscle is isolated from the ventricular muscle by a continuous fibrous barrier. This barrier usually provides insulation, preventing the cardiac impulse from passing between atrial and ventricular muscle through some other route other than forwarding conduction via the A-V bundle. (On rare occasions, an irregular muscle bridge does cross the fibrous barrier anywhere other than the A-V bundle.) In such cases, the cardiac impulse will re-enter the atria from the ventricles, resulting in severe cardiac arrhythmias.)
Distribution of Right and Left Branch of Purkinje Fiber in the Ventricles
The distal part of the A-V bundle travels downward in the ventricular septum for 5 to 15 millimeters towards to apex of the heart after entering the fibrous tissue between all the atrial and ventricular muscles. The bundle, therefore, divides into left and right bundle branches, which lie underneath the endocardium on opposite sides of the ventricular septum. Each branch extends downward toward the ventricle’s apex, gradually splitting into smaller branches. These branches, in turn, wind their way sideways around each ventricular chamber and back toward the heart’s base. The Purkinje fiber penetrates about one-third of the way into the muscle mass before joining the cardiac muscle fibers.
From the moment the cardiac impulse enters the bundle branches in the ventricular septum until it reaches the Purkinje fiber terminations, the cumulative elapsed time is just 0.03 seconds. As a result, when a cardiac impulse reaches the ventricular Purkinje conductive system, it almost inevitably runs through the entire ventricular muscle mass.
Cardiac Impulse Transmission into the Ventricular Muscles
The impulse is mediated through all the ventricular muscle mass by the ventricular muscle fibers directly until it reaches the endings of the Purkinje fibers. The transmission speed is now just 0.3 to 0.5 m/sec, one-sixth of the Purkinje fibers.
Since the cardiac muscle coils around the heart in a double spiral, with fibrous septa between the spiraling sheets, the cardiac impulse does not always move immediately outward toward the surface of the heart but rather angulates toward the surface along lines of the spirals. Because of this angulation, propagation from the endocardial surface of the ventricle to the epicardial surface of the ventricle will take up to 0.03 seconds longer, roughly equal to the time taken for transmission across the entire ventricular portion of the Purkinje system. Thus, in the normal heart, the total time for propagation of the cardiac impulse from the main bundle branches to the last of the ventricular muscle fibers is around 0.06 seconds.
Summary of the Spread of Cardiac Action Potential Through the Heart
The propagation of the cardiac impulse through the human heart is depicted in this image. The numbers on the diagram show the time intervals, in fractions of a second, between the onset of the cardiac impulse in the sinus node and its arrival at each point in the heart. The impulse travels at a moderate speed through the atria but is delayed by more than 0.1 seconds in the A-V nodal area before reaching the ventricular septal A-V bundle.
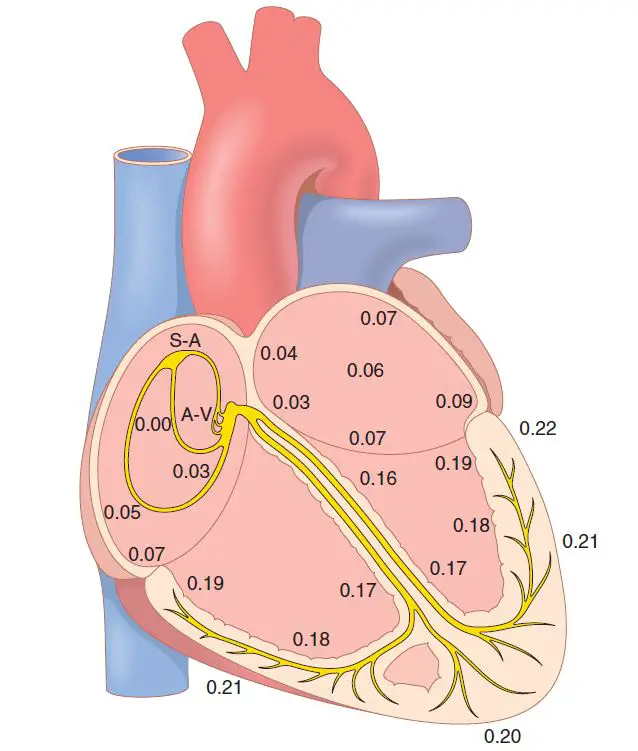
Once within, it spreads quickly through the Purkinje fibers to the full endocardial surface of the ventricles. The impulse then travels slightly more slowly across the ventricular muscle to the epicardial surfaces.
We must understand the path of the cardiac impulse through the heart and the exact times at which it appears in each separate section of the heart; a detailed quantitative understanding of this process is needed for understanding electrocardiography.
Control of Excitation and Conduction of the action potential
The Natural Pacemaker – SA Node
So far in our discussion of the origins and propagation of the cardiac impulse through the heart, we’ve established that the impulse usually originates in the SA node. This is not the case in certain unusual circumstances. Other sections of the heart, such as the A-V nodal and Purkinje fibers, will similarly exhibit intrinsic rhythmical excitation that the SA nodal fibers do.
When not stimulated by an external source, the A-V nodal fibers discharge at an intrinsic(in a healthy individual) rhythmical rate of 40 to 60 times per minute, and the Purkinje fibers discharge at a rate between 15 and 40 times per minute. These rates contrast with the sinus node’s usual rate of 70 to 80 times per minute.
So, why is it that the SA node, rather than the A-V node or the Purkinje fibers, regulates the heart’s rhythm?
The response stems from the fact that the SA node’s discharge rate is significantly faster than the natural self-excitatory discharge rate of either the A-V node or the Purkinje fibers. Like the SA node discharges, the impulse is conducted through both the A-V node and the Purkinje fibers, causing their excitable membranes to discharge as well. However, the SA node discharges again before the A-V node or the Purkinje fibers can meet their self-excitation thresholds.
As a result, before self-excitation can occur in any of these locations, the new impulse from the SA node discharges both the A-V node and the Purkinje fibers.
As a result, the SA node regulates the heartbeat because its rate of rhythmical discharge is higher than that of any other part of the heart. As a result, the sinus node is almost always the usual heart’s pacemaker.
Ectopic Pacemaker – Abnormal Condition of the Heart
Sometimes, any part of the heart develops a faster rhythmical discharge rate than the SA node. This can happen in the A-V node or the Purkinje fibers, for example, if either of these becomes abnormal. In any case, the heart’s pacemaker moves from the SA node to the A-V node or the excited Purkinje fibers. In rare cases, a location in the atrial or ventricular muscle becomes too excitable and becomes the pacemaker.
An “ectopic” pacemaker is located somewhere other than the SA node. An ectopic pacemaker induces an irregular series of contractions of the various sections of the heart which may result in severe heart pumping debility.
Another source of pacemaker change is a blockage in the propagation of the cardiac impulse from the SA node to the rest of the heart. The new pacemaker is then most often found at the A-V node or in the penetrating section of the A-V bundle on its way to the ventricles.
Usually, A-V block takes place, when the cardiac impulse fails to transmit naturally from the atria into the ventricles through the A-V nodal and bundle system—the atria continue to beat at the SA node’s regular rate of rhythm, while a new pacemaker normally develops in the Purkinje system of the ventricles and maintains the ventricular muscle at a new rate of 15 to 40 beats per minute. The Purkinje fiber system does not begin to emit its intrinsic and natural rhythmical impulses until 5 to 20 seconds after the A-V bundle blockage because the Purkinje fibers were “overdriven” by the rapid SA impulses before the blockage and are thus suppressed. The ventricles fail to pump blood during these 5 to 20 seconds, and the patient or victim faints for the first 4 to 5 seconds due to a lack of blood flow to the brain consequently lack of glucose.
This condition is known as Stokes-Adam syndrome; this syndrome refers to the delayed detection of the pulse. If the delay is too long, it may result in death.
Role of Purkinje Fiber
The Purkinje system’s rapid conduction usually allows the cardiac impulse to reach almost all parts of the ventricles in a short period, exciting the first ventricular muscle fiber just 0.03 to 0.06 seconds before excitation of the last ventricular muscle fiber. Because of this timing, all parts of the ventricular muscle in both ventricles begin contracting at nearly the same time and keep contracting for about 0.3 seconds.
This synchronous form of contraction is needed for effective pumping by the two ventricular chambers. If the cardiac impulse travels slowly through the ventricles, most of the ventricular mass will contract before the remainder, resulting in a greatly reduced overall pumping effect.
Slow propagation does occur in certain forms of cardiac debilities, and the pumping efficiency of the ventricles is reduced by up to 30%.
Role of Sympathetic and Parasympathetic Nerve in Cardiac Impulse
Both sympathetic and parasympathetic nerves supply the heart. The parasympathetic nerves (the vagi) are mostly allocated to the S-A and A-V nodes, with a minor distribution to the muscle of the two atria and very little specifically to the ventricular muscle. The sympathetic nerves, on the other hand, are distributed throughout the heart, with a good representation of the ventricular muscle as well as all other regions.
Role of Parasympathetic Nerve (Vagal)
The hormone acetylcholine is released at the vagal ends when the parasympathetic nerves to the heart (the vagi) are stimulated.
This hormone has two important effects on the cardiovascular system. It slows the propagation of the cardiac impulse into the ventricles by first decreasing the rate of the rhythm of the SA node and then decreasing the excitability of the A-V junctional fibers between the atrial musculature and the A-V node.
Weak to medium vagal stimulation reduces the rate of heart-pumping to 1/2 of normal.
Besides that, extreme stimulation of the vagi can simply stop the sinus node’s rhythmical excitation or restrict the transmission of the cardiac impulse from the atria to the ventricles via the A-V node.
Rhythmical excitatory impulses are no further transmitted into the ventricles in either situation. The ventricles may stop beating for 5 to 20 seconds, and somehow a particular section in the Purkinje fibers, usually in the ventricular septal portion of the A-V bundle, establishes its rhythm and induces ventricular contraction at a rate of 15 to 40 beats per minute. This is referred to as ventricular escape.
Mechanism of Vegal action
Acetylcholine generated at vagal nerve endings significantly increases the permeability of fiber membranes to potassium ions, allowing potassium to leak rapidly out of the conductive fibers. This process induces increased negativity within the fibers, a phenomenon known as hyperpolarization, which reduces the excitability of this excitable tissue.
The condition of hyperpolarization in the sinus node causes the “resting” membrane potential of the sinus nodal fibers to be significantly more negative than average, that is, -65 to -75 millivolts instead of the typical range of -55 to -60 millivolts.
As a result, the initial increase in the sinus nodal membrane potential induced by internal sodium and calcium leakage takes much longer to meet the excitation threshold potential. This necessity significantly delays the rhythmicity of these nodal fibers. If the vagal stimulation is high enough, the rhythmical self-excitation of this node can be fully stopped.
A condition of hyperpolarization in the A-V node triggered by vagal stimulation makes it extremely difficult for the tiny atrial fibers entering the node to produce enough electricity to excite the nodal fibers. As a result, the protection factor for cardiac impulse transmission through transitional fibers into A-V nodal fibers reduces.
A small decrease merely slows impulse conduction, while a massive decrease completely blocks conduction.
Role of Sympathetic Stimulation for increasing the Cardiac Impulse
The effects of sympathetic stimulation on the heart are the opposite of those of vagal stimulation, as follows:
- It raises the rate of SA nodal discharge.
- It raises the rate of conduction as well as the degree of excitability in the heart.
- It significantly increases the force of contraction of all cardiac musculature, both atrial and ventricular.
In a nutshell, sympathetic stimulation raises the heart’s overall function. Maximal stimulation can nearly triple the frequency of heartbeats and increase the strength of heart contractions by up to twofold.
Mechanism of Sympathetic stimulation in heart
The hormone norepinephrine is released at sympathetic nerve endings when the sympathetic nerves are stimulated. In exchange, norepinephrine activates beta-1 adrenergic receptors, which shows the impact on heart rate. Although the exact mechanisms by which beta-1 adrenergic stimulation works on cardiac muscle fibers is unknown, it is suggested to enhance the permeability of the fiber membrane to sodium and calcium ions. A rise in sodium-calcium permeability induces a more positive resting potential in the SA node, as well as an increase in the rate of upward migration of the diastolic membrane potential toward the threshold value for self-excitation, thereby accelerating self-excitation and, as a result, increasing the heart rate.
A rise in sodium-calcium permeability induces a more positive resting potential in the SA node, as well as an increase in the rate of upward migration of the diastolic membrane potential toward the threshold value for self-excitation, thereby accelerating self-excitation and, as a result, increasing the heart rate.
Elevated sodium-calcium permeability in the A-V node and A-V bundles makes it much easier for the action potential to excite each subsequent portion of the conducting fiber bundles, reducing conduction time from the atria to the ventricles.
Since calcium ions play a crucial role in exciting the contractile mechanism of the myofibrils, the increase in permeability to calcium ions is at least partially responsible for the high contractile strength of the cardiac muscle under the effect of sympathetic stimulation.
Read Venous Return
Few Last Words
The cardiac action potential is not hard to understand. Hope the discussions help you.
Last Updated on February 23, 2022 by Learn From Doctor Team